The human brain is comprised of thousands of unique types of neurons, each with distinct roles and developmental pathways. This intricate neuronal diversity serves as the foundation for our cognitive abilities and behaviour.
Professor Geoff Faulkner and his lab at UQ’s Queensland Brain Institute study the genetic factors responsible for this neuronal diversity. Specifically, the lab aims to understand how mobile genes are expressed in neurons, impact their activity, and cause dysfunction, shifting the brain from a healthy state to a diseased state.
Dr Gabriela-Oana Bodea is a senior research fellow in the Faulkner lab. Dr Bodea has been investigating a specific type of mobile genes, called retrotransposons, linked to several neurological diseases, including schizophrenia and Parkinson’s disease.
Dr Bodea, you research the role of retrotransposons, a specific type of mobile genes in neurons. Can you tell us more about these ‘jumping genes’?
Retrotransposons are mobile genes that can create copies of themselves and insert these copies at new locations within the genome. Where they insert themselves is somewhat random – there is no exact point or locus that they move to. While we know that these genes can jump around in the brain, it is unclear what their purpose is.
We have been studying one retrotransposon called Line 1 (L1). There are hundreds of thousands of copies of L1 in our genomes, making up almost 20 percent of our DNA. Only 100 or so copies are active and can move around.
While L1 activity in neurons was discovered over a decade ago, the extent and specificity of this activity, as well as its impact on brain cell function, has remained unclear.
Do these L1 elements jump around the genome throughout our lives?
In people, mobile genes make up approximately 50% of the genome. While most L1s are inactive, the small number that are active can jump throughout an individual’s lifetime. This activity is more common in early embryos and certain tissues like the brain. L1 mobilisation in germline can be inherited, contributing to genetic diversity and evolution. L1 jumping in somatic cells can lead to genetic mosaicism within an individual.
When L1s land somewhere in the genome, they can insert themselves into important genes, disrupting function and wreaking havoc on neurons.
Does the activity of L1 genes vary between different neuronal cell types?
Current research suggests that the genomic distribution and activity of L1s may vary between different types of neurons. While most L1 copies in neurons are inactive, there is variability in active L1s among neuronal populations.
There is a specific type of neurons, called parvalbumin (PV) interneurons, which are involved in maintaining the balance between neuronal excitation and inhibition, and play a significant role in memory regulation. These neurons are highly sensitive to stressors and their dysfunction has been implicated in many neuropsychiatric diseases. We found that L1 is active in PV interneurons more than other neurons and it may play a role in their development and function. Further research is needed to determine the precise mechanisms by which L1 activation affects neuronal function.
Understanding the role L1 plays on neuronal function is a step toward developing therapies to protect these neurons in various disease states.
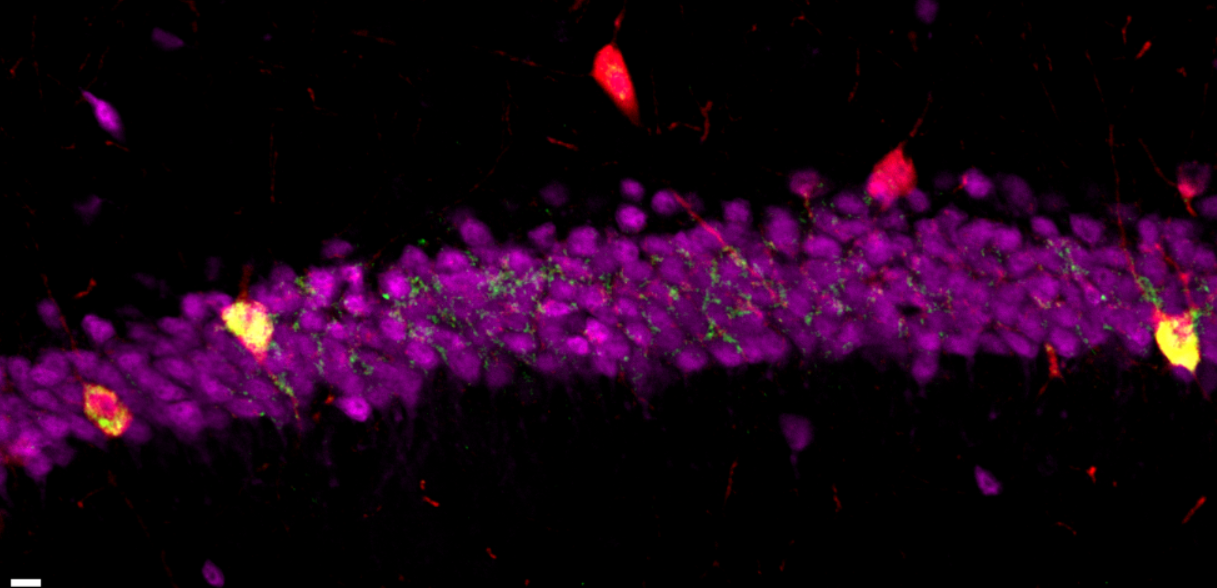
This has been a long research project that has yielded significant findings. How have technological advances assisted in getting to this point?
Yes, we have been working on this research for over 8 years, but only recently have we had the experimental tools to significantly advance our knowledge.
L1s are relatively long sequence of DNA that have been challenging to analyse with traditional sequencing methods.
Recent advances in nanopore sequencing technology have enabled us to sequence these and other mobile genes, more accurately and much faster. Using this new sequencing technique, we were able to sequence L1sand identify specific modifications within their DNA sequence.
This research links retrotransposons to neuronal diversity, and we think that the extent of this diversity may be a fundamental aspect of healthy brain function. Understanding the foundations of this diversity is significant for understanding functioning in a healthy brain so that later we can develop approaches to prevent dysfunction in brain disease.
This research was published in Nature Neuroscience.