How zebrafish can help the science of neurodegeneration
A 21-day-old zebrafish. (Credit: Wikicommons)
Zebrafish (Danio rerio) are probably not the first creatures that come to mind when it comes to animals that are valuable for medical research.
You might struggle to imagine you have much in common with this small tropical freshwater fish, though you may be inclined to keep a few 'zebra danios' in your home aquarium, given they are hardy, undemanding animals that cost only a few dollars each.
Yet each year more and more scientists are turning to zebrafish to unravel the mechanisms underlying genetic or infectious diseases, from muscular dystrophy, to MND, schizophrenia, tuberculosis or cancer.
So what is it about zebrafish that has taken them from the freshwater rivers and streams of Southeast Asia, beyond the pet shops and into universities and research institutes the world over?
A short history of zebrafish
A scientist called George Streisinger, working at the University of Oregon in Eugene, USA in the 1970s and 80s, recognised the vast potential of this organism for developmental biology and genetics research.
In contrast to fruit flies and worms, the other simple model organisms established at the time, zebrafish are vertebrates.
They have a backbone, brain and spinal cord as well as several other organs, including a heart, liver and pancreas, kidneys, bones and cartilage, which makes them much more similar to humans than you may have otherwise thought.
But as a vertebrate model, could they be as useful as mice?
Several things captured Streisinger’s imagination.
Most famously, zebrafish embryos, unlike mouse embryos, develop outside the mother’s body and are transparent throughout the first few days of life.
This provides unparallelled opportunities for researchers to scrutinise the fine details of embryonic vertebrate development without first having to resort to invasive procedures or euthanising the mother.
But this advantage is enhanced by the fact zebrafish reproduce profusely (each pair can produce 200-300 fertilised eggs every week); an ideal attribute for genetic studies. Again, the large, external embryos are a critical part of this success.
When just one or two cells old, zebrafish embryos can be easily microinjected with mRNA or DNA corresponding to genes of interest; undeterred, they then they go on to grow and reproduce, handing down the injected gene to the next generation.
From zebrafish to humans
A paper published in Nature unveiled the long-awaited sequence of the zebrafish genome, revealing that zebrafish, mice and human have 12,719 genes in common.
Put another way, 70% of human genes are found in zebrafish. But even more notable is the finding that 84% of human disease-causing genes are found in zebrafish.
Perhaps not surprisingly then, when these genes are injected into zebrafish embryos, the growing animals are doomed to acquire the same diseases.
And while zebrafish are still used widely to answer fundamental questions of developmental biology, much current research is directed towards combining their many attributes in studies that are designed to improve human health.
This is especially true for cancer research where the expression of cancer-causing genes (oncogenes) can be directed to specific organs, virtually at will.
This process, known as transgenesis, is very straightforward in zebrafish and has allowed researchers to produce zebrafish models of liver, pancreatic, skeletal muscle, blood and skin cancers, to name but a few.
And when the genomic make-up of these zebrafish tumours is deciphered using the latest DNA sequencing technology, the patterns of mutations, or “gene signatures”, are found to overlap substantially with those in the corresponding human tumours.
Tumour growth
As well as the transgenic zebrafish models of cancer, researchers are also transplanting cells derived from human tumours into zebrafish embryos and watching them grow and spread.
Coupled with the advent of high resolution live-imaging techniques, the birth, growth and spread of tumours can be scrutinised in movies that can be played over and over again.The creation of a transparent (non-striped) version of adult zebrafish (called Casper, after the cartoon ghost) means the behaviour of tumour cells inside these living organisms can be followed for days at a time.
These experiments are usually conducted in zebrafish that have been genetically modified to express genes that glow in specific body compartments, giving researchers the ability to pinpoint potentially critical connections between “host” cells and tumour cells that may determine whether the latter survive or die.
This type of experiment is revealing a complex interplay of potentially beneficial and detrimental components.
While the proximity of immune cells may instigate mechanisms capable of destroying the tumour, the stimulation of new blood and lymphatic vessel growth towards the tumour is more insidious, since it delivers the tumour with both the nutrients it needs to survive and a network to spread throughout the body.
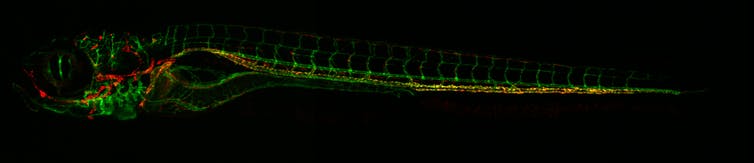
These processes, once properly understood, are likely to provide opportunities for therapeutic intervention in the future.
Zebrafish research
Cancer research is just one part of the zebrafish story. In Australia alone, investigators are also using zebrafish to study metabolic disorders such as:
- diabetes
- muscle diseases, including muscular dystrophy
- neurodegenerative diseases
- neuron communication
- the response of the host innate immune system to bacterial and fungal infections
Excitingly, research is also underway in this country to unravel the genetic mechanisms controlling heart, skeletal muscle and nervous tissue regeneration in zebrafish, in the hope that these processes can be one day transferred to humans to address the burgeoning socioeconomic problem of tissue degeneration in our ageing population.
So next time you peer into someone’s home aquarium, imagine the biomedical possibilities inherent in this lively and amiable little fish!
Joan Heath, Laboratory Head, Walter and Eliza Hall Institute, and Associate Professor of Medical Biology, University of Melbourne
This article was originally published on The Conversation. Read the original article.